Design and construction of a GAP-43 reporter system for potential identification of effective therapeutics for peripheral nerve regeneration
DOI:
https://doi.org/10.33178/SMJ.2023.1.2Keywords:
peripheral nerve injury, nerve regeneration, regeneration associated genes, GAP-43Abstract
Background: Peripheral nerve injury (PNI) is a condition that can result in muscle paralysis and sensory disturbances. Electrical stimulation and/or the application of exogenous neurotrophic factors and cytokines are effective at enhancing nerve regeneration and is mediated via the expression of regeneration associated genes (RAGs) such as the growth associated protein GAP-43. Therapeutic upregulation of GAP-43 has potential use as a treatment for improving recovery from PNI. Few studies have investigated the potential of increasing GAP-43 for PNI therapeutic purposes and current methods for measuring GAP-43 expression are limited.
Aims and Objectives: The broader aim of this work was to construct a motor neuron-like cell model with a GAP-43 reporter system. Such a model would have potential use in screening for novel therapeutics that upregulate GAP-43 and in the optimisation of electrical stimulation treatment in combination with these therapies.
The key aim of the work was to design and construct a Cas9 expressing plasmid bearing a gRNA that targets GAP-43 cleavage and a donor plasmid bearing a reporter GFP or Neo cassette flanked with 5’ and 3’ GAP-43 homology arms (HAs) to facilitate the insertion of the reporter immediately 3’ of the GAP-43 promoter via CRISPR/Cas9 homology directed repair (HDR). Such an insertion would enable quantitative measurement of endogenous expression of the GAP-43 gene.
Methods and Results: To guide the Cas9 nuclease to the target location, GAP-43 gRNA oligomers were designed and cloned downstream of the U6 promoter in the Cas9 expression plasmid px330, which also expresses the Cas9 gene and the cloned gRNA when transfected into cells.
For CRISPR/Cas9 HDR, the 5’ and 3’ GAP-43 HAs were amplified from mouse genomic DNA and cloned into the donor plasmid using Gibson Assembly so that they flanked the reporter cassette.
Results: This work successfully constructed the Cas9 gRNA expressing plasmid to target cleavage of the GAP-43 gene in mouse cell lines and has provided the complete design and construction foundation for generation of the reporter system for the endogenous GAP-43 gene in mice.
References
Huckhagel T, Nüchtern J, Regelsberger J, Gelderblom M, Lefering R. Nerve trauma of the lower extremity: evaluation of
,422 leg injured patients from the TraumaRegister DGU® between 2002 and 2015. Scandinavian Journal of Trauma,
Resuscitation and Emergency Medicine [Internet]. 2018 [cited 27 October 2021];26(1). Available from: https://www.scopus.
com/record/display.uri?eid=2-s2.0-85047079475&origin=inward
Zuo K, Gordon T, Chan K, Borschel G. Electrical stimulation to enhance peripheral nerve regeneration: Update in molecular
investigations and clinical translation. Experimental Neurology [Internet]. 2020 [cited 27 October 2021];332:113397.
Available from: https://www.sciencedirect.com/science/article/abs/pii/S0014488620302284?via%3Dihub
de Medinaceli L, Seaber A. Experimental nerve reconnection: Importance of initial repair. Microsurgery [Internet]. 1989
[cited 27 October 2021];10(1):56-70. Available from: https://onlinelibrary.wiley.com/doi/10.1002/micr.1920100111
Seddon HJ. Three types of nerve injury. Brain. 1943 Dec 1;66(4):237-88.
LoPachin R, Lehning E. Mechanism of Calcium Entry during Axon Injury and Degeneration. Toxicology and Applied
Pharmacology. 1997;143(2):233-244.
Yoo S, Nguyen MP, Fukuda M, Bittner GD, Fishman HM. Plasmalemmal sealing of transected mammalian neurites is a
gradual process mediated by ca2+-regulated proteins. Journal of Neuroscience Research. 2003;74(4):541–51.
Mata, M., Staple, J. and Fink, D., 1986. Changes in intra-axonal calcium distribution following nerve crush. Journal of
Neurobiology, 17(5), pp.449-467.
Al-Majed A, Tam S, Gordon T. Electrical Stimulation Accelerates and Enhances Expression of Regeneration-Associated
Genes in Regenerating Rat Femoral Motoneurons. Cellular and Molecular Neurobiology. 2004;24(3):379-402.
McGregor, C. and English, A., 2019. The Role of BDNF in Peripheral Nerve Regeneration: Activity-Dependent Treatments
and Val66Met. Frontiers in Cellular Neuroscience, [online] 12. Available at: <https://www.frontiersin.org/articles/10.3389/
fncel.2018.00522/full#B175> [Accessed 10 November 2021].
van Kesteren R, Mason M, MacGillavry H, Smit A, Verhaagen J. A Gene Network Perspective on Axonal Regeneration.
Frontiers in Molecular Neuroscience [Internet]. 2011 [cited 27 October 2021];4. Available from: https://www.frontiersin.
org/articles/10.3389/fnmol.2011.00046/full
Chung D, Shum A, Caraveo G. GAP-43 and BASP1 in Axon Regeneration: Implications for the Treatment of
Neurodegenerative Diseases. Frontiers in Cell and Developmental Biology. 2020;8.Available from: https://www.frontiersin.
org/articles/10.3389/fcell.2020.567537/full
Mandal K. Review of PIP2 in Cellular Signaling, Functions and Diseases. International Journal of Molecular Sciences
[Internet]. 2020 [cited 20 November 2021];21(21):8342. Available from: https://www.ncbi.nlm.nih.gov/pmc/articles/
PMC7664428/
Eggen B, Nielander H, Rensen-de Leeuw M, Schotman P, Gispen W, Schrama L. Identification of two promoter regions in
the rat B-50/GAP-43 gene. Molecular Brain Research [Internet]. 1994 [cited 20 November 2021];23(3):221-234. Available
from: https://pubmed.ncbi.nlm.nih.gov/8057779/
de Groen P, Eggen B, Gispen W, Schotman P, Schrama L. Cloning and promoter analysis of the human B-50/GAP-43 gene.
Journal of Molecular Neuroscience [Internet]. 1995 [cited 20 November 2021];6(2):109-119. Available from: https://
pubmed.ncbi.nlm.nih.gov/8746449/
Nedivi E, Basi G, Akey I, Skene J. A neural-specific GAP-43 core promoter located between unusual DNA elements that
interact to regulate its activity. The Journal of Neuroscience [Internet]. 1992 [cited 20 November 2021];12(3):691-704.
Available from: https://pubmed.ncbi.nlm.nih.gov/1532026/
Allegra Mascaro, A., Cesare, P., Sacconi, L., Grasselli, G., Mandolesi, G., Maco, B., Knott, G., Huang, L., De Paola, V., Strata,
P. and Pavone, F., 2013. In vivo single branch axotomy induces GAP-43-dependent sprouting and synaptic remodeling in
cerebellar cortex. Proceedings of the National Academy of Sciences, [online] 110(26), pp.10824-10829. Available at:
www.pnas.org/content/110/26/10824.short> [Accessed 20 December 2021]
Skene JH, Willard M. Axonally transported proteins associated with axon growth in rabbit central and Peripheral Nervous
Systems [Internet]. The Journal of cell biology. The Rockefeller University Press; 1981 [cited 2021Dec27]. Available from:
https://www.ncbi.nlm.nih.gov/pmc/articles/PMC2111762/
Aigner L, Arber S, Kapfhammer JP, Laux T, Schneider C, Botteri F, et al. Overexpression of the neural growth-associated
protein gap-43 induces nerve sprouting in the adult nervous system of transgenic mice. Cell. 1995;83(2):269–78.
Finkbeiner S. Calcium regulation of the brain-derived neurotrophic factor gene. Cellular and Molecular Life Sciences.
;57(3):394–401.
Rojas-Fernandez A, Herhaus L, Macartney T, Lachaud C, Hay RT, Sapkota GP. Rapid generation of endogenously driven
transcriptional reporters in cells through CRISPR/Cas9. Scientific Reports. 2015;5(1).
Cong L, Ran FA, Cox D, Lin S, Barretto R, Habib N, et al. Multiplex Genome Engineering using CRISPR/Cas Systems. Science.
;339(6121):819–23.
Thomas KR, Folger KR, Capecchi MR. High frequency targeting of genes to specific sites in the mammalian genome. Cell.
;44(3):419–28.
Clarke L. PCR amplification introduces errors into mononucleotide and dinucleotide repeat sequences. Molecular Pathology
[Internet]. 2001 [cited 23 November 2021];54(5):351-353. Available from: https://www.ncbi.nlm.nih.gov/pmc/articles/
PMC1187094/
Geuna, S., Raimondo, S., Fregnan, F., Haastert-Talini, K. and Grothe, C., 2016. In vitro models for peripheral nerve
regeneration. European Journal of Neuroscience, [online] 43, pp.287-296. Available at: <https://pubmed.ncbi.nlm.nih.
gov/26309051/> [Accessed 22 December 2021].
Maier, O., Böhm, J., Dahm, M., Brück, S., Beyer, C. and Johann, S., 2013. Differentiated NSC-34 motoneuron-like cells
as experimental model for cholinergic neurodegeneration. Neurochemistry International, [online] 62(8), pp.1029-1038.
Available at: <https://pubmed.ncbi.nlm.nih.gov/23562846/> [Accessed 20 November 2021].
Anderson W. Development of a Functional In Vitro 3D Model of the Peripheral Nerve [PhD]. University of Central Florida;
Boyd JG, Gordon T. A dose-dependent facilitation and inhibition of peripheral nerve regeneration by brain-derived
neurotrophic factor. European Journal of Neuroscience. 2002;15(4):613–26.
Hakkoum D, Stoppini L, Muller D. Interleukin-6 promotes sprouting and functional recovery in lesioned organotypic
hippocampal slice cultures. Journal of Neurochemistry. 2007;100(3):747–57.
Cox AA, Sagot Y, Hedou G, Grek C, Wilkes T, Vinik AI, et al. Low-dose pulsatile interleukin-6 as a treatment option for
diabetic peripheral neuropathy. Frontiers in Endocrinology. 2017;8.
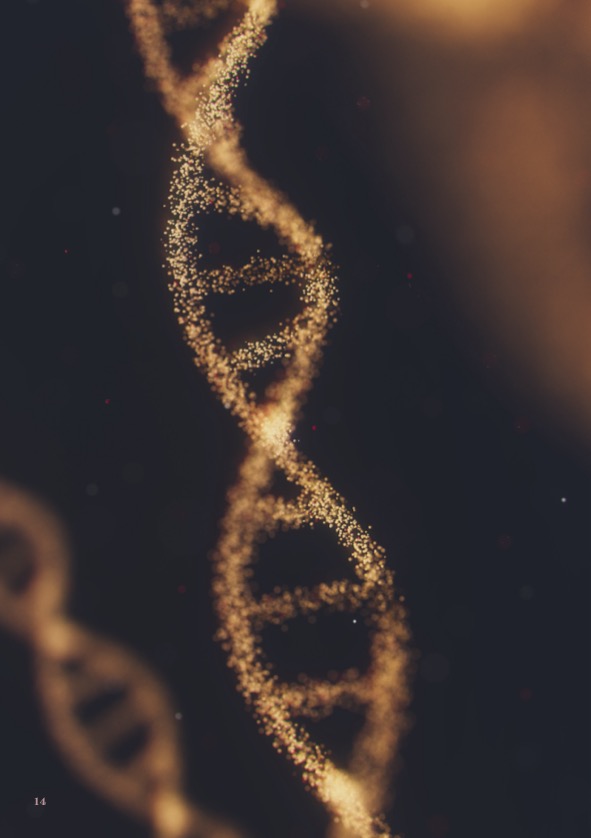
Downloads
Published
License
Copyright (c) 2024 Nina Carlos-De Clercq, Lisa Breen, Tommy McCarthy

This work is licensed under a Creative Commons Attribution-NonCommercial 4.0 International License.